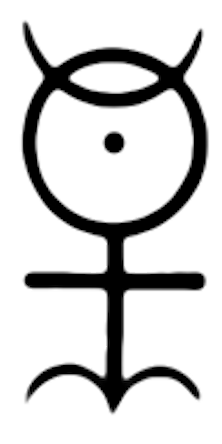
0
There are not items in your cart.
Visit Shop
Product is not available in this quantity.
Polysaccharide is widely distributed in natural resources, and currently attracts scientists' attention for their various bio-functions including immunomodulatory activity, hypoglycemic activity, hypolipidemic activity, antitumor activity, promoting effects on gastrointestinal function, and so on. The purity and structure characteristics of different polysaccharides greatly restrict the in-depth study of their bioactivity mechanism. Therefore, this guideline will display the extraction, isolation, purification, and structural characterization of polysaccharides from natural resources. The extraction methods of acid-soluble polysaccharides, water-soluble polysaccharides, and alkaline soluble polysaccharides are included. Common purification methods like membrane filtration, alcohol precipitation, and column chromatographic separation are explained in detail. A comprehensive procedure for polysaccharide structural characterization is given from molecular weight and monosaccharide composition study to Fourier transform infrared and nuclear magnetic resonance analysis. Examples are added where necessary to help you better understand this guideline.
ASTRA
Polysaccharides are abundant biomacromolecules in nature and are widely found in plants, animals, and microorganisms (fungi and bacteria). In recent years, numerous studies had revealed that polysaccharides from natural sources had various bioactive functions, such as immunoregulation (Sun et al., 2021), regulation of gut microbiota (H. Lin, Zhang, et al., 2021), antitumor (Zhao et al., 2020), anti-inflammatory (J. Zhang et al., 2021), blood sugar and lipid regulation (Y. Zhang et al., 2021), and so on. Its extraction and purification are the first and also one of the most critical parts of complex carbohydrate study. And the structure information is of great importance to uncover its bioactivity mechanism (Wang, Zhang, et al., 2021). In this case, it is necessary to summarize the extraction, separation, purification, and structure analysis procedure of polysaccharides.
In brief, the preparation of polysaccharides includes extraction, separation, and purification. Crude polysaccharide is achieved after water extraction. Separation and purification aim to enrich polysaccharide substances and obtain the polysaccharide fractions (Tang et al., 2020). Impurities like starch, protein, pigment, oligosaccharide, and lipids should be removed before fraction purification. Currently, the chosen purification method is mainly based on its chemical properties. Ion exchange chromatography, hexadecyltrimethylammonium bromide precipitation, gradient ethanol precipitation, salt fractionation, and gel chromatography are frequently used for fraction purification.
The primary structure of a polysaccharide should contain information such as monosaccharide composition, molecular weight, the type of glycosidic bonds in the polysaccharide chain, and the order of connection. Equipment including high-performance liquid chromatography (HPLC), ion exchange chromatography, gas chromatography (GC), gas chromatograph-mass spectrometer (GC-MS), high-performance anion exchange chromatography (HPAEC), and nuclear magnetic resonance (NMR) is required in this process. Since polysaccharides exist in numerous kinds of natural materials, neither the extraction method nor the structure information display differs according to the material types. And you may have read lots of papers on polysaccharide extraction and literature on structure information. In this review, we summarized a general procedure of polysaccharide preparation and structure analysis, and examples are given for each part to help you better understand.
Hot water extraction is the most commonly used method in soluble neutral polysaccharide extraction from plant resources (Yu et al., 2022). The following steps are included in general: material preparation and grinding→lipid removal→water extraction→extracts collection and vacuum concentration→ethanol precipitation→precipitates collection and drying→crude polysaccharide achieved→refining the crude polysaccharide→refined polysaccharide achieved. Details for each procedure are explained as follows.
In addition to intracellular polysaccharides, plant tissues also contain other polysaccharides, such as cellulose, hemicellulose, and pectin. These polysaccharides are intertwined with each other to form a three-dimensional primary wall structure, which is hardly extracted by a hot neutral aqueous solution (Z. Liu et al., 2019). Pectin, a representative acid-soluble polysaccharide which negatively charged in an aqueous solution, exists widely in the cell wall of plants. The theory of using acid for pectin extraction is that the protopectin could be converted to water-soluble pectin in the acidic solution. The acid extraction process of sugar beet pectin is shown below (Ai et al., 2019):
(a) The pretreatment of sugar beet pulp. Five hundred gram sugar beet pulp is rinsed using 1.5 L distilled water three times to remove the impurities. After draining water, the washed pulp is mixed with 1.5 L distilled water, and extracted at 80°C with stirring for removing the remained free sugar. After extraction, the extracted pulp is put in a 400 mesh nylon cloth and squeezed for draining the extract, then, the residue is rinsed using 1 L distilled water three times, and then, flatted on a stainless steel plate and air dried at 45°C for 48 h in a drying oven. Notably, to prevent the fermentation of sugar beet pulp during the air-dried process, the pulp must be stirred evenly once an hour in the early 12 h. The dried pulp is ground to pass through a 20 mesh screen and stored at −20°C in a fridge.
(b) The extraction process of pectin. Forty-gram ground sugar beet pulp is mixed with 1 L distilled water under stirring, and then, pH value of the mixture is adjusted to 1.5 using 5 M H2SO4. The mixture is extracted at 80°C for 1 h in a thermostat water bath with stirring. After extraction, the mixture is filtered through a 400-mesh nylon cloth, and the filtrate is centrifuged at 10,000g for 20 min. After centrifugation, the supernatant is collected and mixed with three volumes of ethanol with stirring, then, settled at ambient temperature for 10 h. The precipitated pectin is separated by centrifugation at 12,000g for 20 min. The resulting pectin is washed twice using 75% ethanol and washed twice using 95% ethanol (at a weight ratio of a solvent-pectin pellet of 3:1), and filtered using a 400 mesh nylon cloth. The collected pectin is air dried at 45°C for 24 h in a drying oven and ground to pass through a 100-mesh screen, and then stored in a dryer. The extraction rate is calculated as below:ET%=C1/C2×100%,(1)where C1 is the weight of initial sugar beet pulp, and C2 is the dried pectin.
The alkaline aqueous solution is usually used for the extraction of hemicellulose. In general, pectin could be also extracted by an alkaline aqueous solution. However, due to the saponification reaction between the alkaline and esterification groups of pectin, the molecular structure of pectin prepared using an alkaline aqueous solution would be changed greatly. Thus, few related works have been reported. In an alkaline aqueous solution, the hydrogen bond among polysaccharides, and the ester bonds and ether bonds among hemicellulose, lignin, pectin, and cellulose would be broken (Brienzo et al., 2009). Particularly, when an alkaline hydroxyl peroxide-based procedure is used, radical reactions will be involved as below:H2O2+HO−→H2O+HOO−(2)
H2O2+HOO−→HO•+O2−•(3)
The alkaline extraction process of alkaline soluble polysaccharides of sugar beet pectin is shown below (Ai et al., 2019):
To sum up the extraction methods, a guideline for choosing the best extraction method could be described as the following: different polysaccharides possessed varied extraction difficulty. The intracellular polysaccharides, such as starch, possessing weak connections with plant tissues, could be easily released by cracking plant cells. However, some polysaccharides, such as pectin, and hemicellulose, are the components of the cell wall. They are covalently linked with other cell wall components (e.g. lignin, cellulose), and hard to be extracted by neutral hot water. Therefore, in the case of different polysaccharides, varied extraction methods are required to achieve satisfactory extract efficiency. Theoretically, extraction using acid or alkaline hot water is more violent and is adequate for extracting the polysaccharides which could be extracted by neutral hot water. However, molecule degradation, such as de-esterification, deprotein, and β-elimination inevitably occurred in the polysaccharides which are placed in strong acid or alkaline medium. Therefore, the extraction method chosen for obtaining polysaccharides should be as mild as possible based on enough extracting efficiency. In other words, the polysaccharide which could be effectively extracted in neutral hot water is better to avoid extracting using acid or alkaline hot water.
Membrane filtration is generally used for juice clarification and concentration, it has been used to concentrate and purify pectin crude aqueous extracts in many studies (Kang et al., 2015). A hollow fiber tangential flow filtration membrane is the most common membrane used in the food industry. The membrane filtration process is shown below (Ai et al., 2020):
Alcohol precipitation is commonly used for purifying polysaccharides from aqueous crude extracts on a laboratory scale (Karnik et al., 2016). Polysaccharides with different polarities are gradually precipitated with the addition of ethanol (or isopropanol) which decreased the dielectric constant of the polysaccharide solution. Due to the high alcohol compatibility, the relatively lower molecular weight sugar components could be removed in this process. Thus, a mixture of polysaccharides could be split into different subfractions by appropriately using alcohol precipitation, based on their different physicochemical properties upon optimizing the processing parameters. The alcohol precipitation is performed as the below step (Ai et al., 2020):
In column chromatographic purification techniques, molecules in a mixture applied onto the surface or into the stationary phase are separated from each other while moving with the aid of a mobile phase. These molecules are separated according to their different molecular characteristics such as total charge, size, and shape. Because of these differences, some components of the mixture stay longer in the stationary phase, and they move slowly in the chromatography system, while others pass rapidly into the mobile phase, and leave the system faster (Coskun et al., 2016). Macroporous adsorption chromatography, ion-exchange chromatography, and gel-permeation chromatography are three commonly used column chromatographic techniques based on mechanisms of surface adsorption, ion exchange, and size exclusion.
Macroporous adsorption resin is a kind of organic polymer copolymer with a macroporous structure. It is a kind of synthetic organic polymer adsorbent. It is mainly polymerized with styrene and divinylbenzene in 0.5% gelatin solution with porogen added. In this system, styrene is the polymerization monomer, divinylbenzene is the crosslinking agent, and toluene and xylene are common porogen. They cross-linked and polymerized with each other to form the porous skeleton structure of macroporous adsorption resin. And because of this porous structure, macroporous resins can be used to separate different phytochemicals. In addition, surface adsorption, surface electricity, or hydrogen bond formation result in its adsorption properties. In general, the nonpolar resin is used for the adsorption of nonpolar organic substances from polar solvents, while the strong polar resin is used for the adsorption of polar solutes from nonpolar solvents. Medium polar adsorption resin can adsorb polar substances not only from nonaqueous media but also from polar solutions. Based on this principle, adsorption resins are applied to the removal of pigments and proteins from crude polysaccharides.
The general separation procedure of macroporous resin separation includes the pretreatment of resin, sample loading, elution, and resin regeneration.
A case is given for practice here. In our previous study, the crude polysaccharides from Red kidney bean and small black bean were purified with JK008 macroporous resin to obtain pure polysaccharides labeled RKP and SBP, respectively. Two grams of crude polysaccharide was dissolved in 40 ml distilled water, and later mixed with 40 g JK008 by magnetic stirrer for 8 h (the ratio of polysaccharide to resin can be multiplied). The miscible liquid was centrifuged at 8000 rpm for 15 min. The supernatant was separated and collected. Finally, the combined supernatant was concentrated by a rotary evaporator and precipitated with quadruple volumes of anhydrous ethanol at 4°C for 8 h to achieve the purified polysaccharide.
Ion exchange chromatography (IEC) is used to separate ionic molecules according to their total charge. Acid polysaccharide has higher interaction intensity with the stationary phase. In this case, acid polysaccharide can be separated from neutral sugar by gradient elution with different ionic strength eluants (Tang et al., 2020). Samples with high viscosity are not suitable for this technique, for the risk to block the column. there are different types of anion exchange resin including DEAE Sepharose Fast Flow, DEAE-cellulose, DEAE-Sephadex, DEAE-Sephadex Fast Flow, Q-Sepharose, and their respective subproducts (Tang et al., 2020).
The general polysaccharide purification with IEC includes resin pretreatment, column balance with buffer, sample loading, elution with solutions with different ion strengths, and collection of different fractions.
A case is given for practice here. A DEAE Sepharose Fast Flow column was applied for the fractionation and purification of tea polysaccharides (Hong et al., 2022). To be specific, Gougunao tea polysaccharide (1 g) was dissolved in 20 ml of distilled water and centrifuged (15,000g, 10 min). The supernatant was applied to a DEAE Sepharose Fast Flow column (Ø45 × 600 mm). The GPS was eluted with distilled water at first, and then with NaCl at different concentrations, successively. Different fractions were collected, concentrated, dialyzed, and freeze-dried, which were labeled as GFw, GPS1, GPS2, GPS3, and GPS4.
The gel is a solid substance coagulated from a colloidal solution. It has a network of sieve holes inside. When the molecules flow through the gel-filled column through the sieve holes in the spherical gel, the macromolecules cannot enter the gel sieve holes. Instead, they only flow through the pores between the gel and the column, and they can soon flow out of the column. Because the smaller molecules enter the sieve holes in the gel, they stay in the column for a long time, thus distinguishing the molecules of different sizes. It can also be compared with molecules of known size to determine the molecular weight of a molecule. At present, the commonly used gels in gel-permeation column chromatography include three main types: dextran gel, agarose gel, and polyacrylamide gel.
Gel column chromatography is suitable for the separation of polysaccharides with different molecular weights. However, due to the mesh inside the gel column filler, if it is a polysaccharide with high viscosity, it is easy to lead to blocking of the column.
The general polysaccharide purification with gel column chromatography includes gel pretreatment and column installation, loading and elution, gel column treatment, and preservation.
A case is given for practice here. DEAE-purified fractions (GPS1, GPS2, GPS3, and GPS4) as described in Section 3.3.2.2 are not homogeneous. Thus, further purification of these fractions was performed by a gel permeation column (Hong et al., 2022). GPS1, GPS2, GPS3, and GPS4 were redissolved in 0.1 M NaCl and further purified on a High-Resolution Sephacryl™ S-400 column (Ø26 × 600 mm, Cytiva). This purification process was monitored by the refractive index detector (RID) (RI-102, SHOKO).
Salt fractionation is a common separation method that separates polysaccharides by different solubilities in the same salt solution. Inorganic salts with a certain concentration added to the polysaccharide solutions induce polysaccharide precipitation due to the different solubility of polysaccharides with different Mw in salt solutions (Hu et al., 2018).
The salt fractionation was generally operated according to the following procedure: Adding neutral salt solution with a certain concentration to the polysaccharide solution, the polysaccharide with larger Mw has a smaller solubility at this concentration and precipitates out first, whereas the polysaccharide with smaller Mw is still retained in the supernatant, accompanied by an increase of salt solution continuously to a higher concentration, the polysaccharide with smaller Mw precipitates out. Common inorganic salts include NaCl, KCl, MgSO4, and (NH4)2SO4, of which (NH4)2SO4 has the best effect on precipitation.
Notably, salt fractionation is cost-effective, but low-efficiency and the polysaccharide precipitates obtained contain many salts that must be removed by dialysis or ultrafiltration. In addition, polysaccharides with high concentration are prone to coprecipitation easily during salinization, consequently, the concentration, pH, and temperature of polysaccharide solution should be carefully controlled to get a satisfactory result. Overall, this method is usually only suitable for separating polysaccharide solutions with a relatively wide molecular weight distribution.
The long chain structure of some polysaccharides may contain some special substitution groups such as carboxyl groups, sulfate groups, and so on, which grant special chemical properties to the polysaccharides and could further be used to precipitate them out from the solvent by simple chemical reactions. Quaternary ammonium precipitation is one of the common chemical precipitation methods (W. Zhang et al., 2016). According to a prior study, quaternary ammonium salt could form coordination by compounding with acidic polysaccharides or polysaccharides of long-chain high Mw in aqueous solutions of low ionic strength (Lei et al., 2016). The acidic polysaccharides or polysaccharides with long-chain high Mw were precipitated first, while neutral fractions were kept in the supernatant. The commonly used quaternary ammonium salts are cetyltrimethylammonium bromide (CTAB) and its base (CTA-OH) and cetyl pyridine (CPC).
The procedure of the quaternary ammonium precipitation is generally followed: The polysaccharide solution is deproteinated, and then a certain volume ratio of quaternary ammonium salt solution is added to form a coordination compound. Based on the solubility of the mixed salt solution and acid solution, as well as an organic solvent with different ionic strengths, the mixed solution is often precipitated by 3–4 mol/L NaCl solution, and then the polysaccharide is precipitated by adding three-five times ethanol while the quaternary ammonium salt remains in the solution. On the other hand, quaternary ammonium salt could be precipitated by adding iodide or potassium thiocyanide salt into 3–4 mol/L NaCl solution, while the polysaccharide remains in the solution. The quaternary ammonium salt can also be extracted by organic solvents, such as n-butanol, pentanol, or chloroform, while the polysaccharide is obtained after dialysis and freeze-drying.
In summary, this method is simple and easy, especially for the separation of acidic and neutral crude polysaccharides. Moreover, complex acidic polysaccharides can be separated by adjusting the concentration of quaternary ammonium salt. However, the yield of polysaccharides is low and the pH must be controlled strictly lower than 9, to protect the neutral polysaccharides from being precipitated out.
The metal complexing method is another common method used for polysaccharide separation. The separation process is based on the precipitation by a complex of polysaccharides and metal ions such as Cu, Ba, and Ca, which will be washed with water sufficiently, and then the purified polysaccharide will be regained by treating with inorganic acid ethanol solution or hydrogen sulfide.
The most commonly used method of metal complexing in the purification of polysaccharides are copper salt complexation and barium hydroxide complexation, such as CuCl2, CuSO4, Cu(Ac)2 solution or Fehling reagent (Chaves et al., 2019). First, the complexing agent solution is added to the polysaccharide solution with stirring, and then obtain the complex precipitation. Notably, the complexing agent solution, except for the Fehling reagent, needs to be used in excess, otherwise, the resulting precipitate will be redissolved. The complex precipitate is washed with water and then decomposed with an ethanol solution of 5% HCl (V/V) or hydrogen sulfide, subsequently washed with ethanol to remove the excess copper salts.
As a whole, based on simple chemical reactions, this type of separation method is effective and suitable for the separation of most crude polysaccharides, but the yield is low. Also, the degree of reaction needs to be strictly controlled during the separation process, to avoid irreversible changes in the structure of polysaccharides that may affect their physiological activity.
Preparative gel electrophoresis is a method of separating, purifying, and preparing biological samples, which is based on the differences of the separated objects, producing different rates of electromigration. Polysaccharides are dissociated and charged in a buffer system at a certain pH, and the charged ions moved directionally under the action of an electric field. According to the different Mw, shapes, and charges of polysaccharides, the migration rates are different under the action of the electric field.
The carrier for preparative gel electrophoresis is usually glass powder, which is mixed into a slurry with water and loaded into a column, subsequently equilibrated with electrophoresis buffer (e.g., 0.05 M borax, pH 9.3) for 3 days. The sample containing crude polysaccharides is placed on the upper end of the column with the power turning on, the upper end is positive (the general direction of electrophoresis of polysaccharides is toward the negative end due to electroosmosis). This electrophoresis column must have a cooling jacket, of which the electric current and voltage per cm are about 30–35 mA and 1.2–2 V, respectively, where electrophoresis lasts about 5–12 h. After electrophoresis, the glass powder carrier is pushed out of the column, split, and eluted separately.
In summary, electrophoresis is commonly applied to the qualitative analysis of polysaccharides due to its better separation effect, but it is only suitable for identifying the purity of polysaccharides and small-scale laboratory application in consequence due to the longer time, small separation amount, and high astriction of the equipment.
Generally, the primary structure of polysaccharides requires the following information: monosaccharide composition, types and linkages of glycosidic bonds, anomeric configuration, and connection sequence of sugar residues (Cui et al., 2005). Figure 1summarizes the methods of polysaccharide structure analysis and the corresponding information.
The molecular weight of polysaccharides is usually determined by high-performance size-exclusion chromatography (HPSEC). Basically, the HPSEC used for the measurement of the molecular weight of polysaccharides should be equipped with a refractive index detector. Furthermore, several detectors, such as photodiode array detector and multiangle light scattering detector, could be combined with HPSEC for enhancing the detection accuracy and detection of the structural elements of polysaccharides. The measurement process is shown below (Ai et al., 2019; J. Lin et al., 2020):
A quantity of 5.00 mg of 10 monosaccharide standards (d-mannose, l-rhamnose, d-arabinose, d-xylose, d-galactose, d-glucose, l-fucose, glucuronic acid standard, d-glucuronic acid, and d-galacturonic acid) are weighed separately and dissolved in 50 ml distilled water. Then the solution is diluted to 10, 8, 6, 4, 2, 1, 0.5, and 0.25 μg/ml, respectively, and submitted to high-performance anion-exchange chromatography with pulsed amperometric (HPAEC-PAD) to form the standard curve of monosaccharide composition analysis.
A quantity of 5 mg polysaccharide sample is hydrolyzed in 12 M H2SO4 in an ice water bath for 30 min and then diluted into 2 M H2SO4 at 100°C for 2 h. After cooling, the hydrolysate was diluted and then submitted to HPAEC-PAD (X. Liu et al., 2017).
The chromatographic conditions are described as follows: Dionex Carbo Pac™ PA 20 (3 mm × 150 mm) is the analytical column, connecting in series with a protective column of Dionex Carbo Pac™ PA 20 (3 mm × 30 mm). The column temperature is 30°C. The injection volume is set to 10 μl, and the temperature of instrument components is 25°C. The mobile phase and gradient elution procedure are shown in Table 1 (Wang et al., 2017). The flow rate of the mobile phase is 0.5 ml/min. The gold electrode is used as a working electrode in an amperometric detector, with Ag/AgCl as a reference electrode.
Table 1. Gradient elution procedure for separation of monosaccharide and uronic acid based on Dionex systemTime (min)0.020.020.130.030.150.055.0A: 250 mmol/L NaOH (%)0.80.80.80.80.8800.8B: H2O (%)99.299.294.279.279.22099.2C: 1 mol/L NaOAc (%)00552000
The result was expressed as a percentage (%) according to the formula: The percentage of monosaccharide(%)=c/(m/nV),where c is the value of the corresponding standard curve of the diluted sample, m is the exact weight of dried polysaccharide (μg), n is the dilution factor, and V is the sample volume (ml).
The acidic polysaccharide, which contains a high content of uronic acid, affects the degree and efficiency of the methylation procedure (Cui et al., 2005). Therefore, the polysaccharide sample shall be reduced by uronic acid before the methylation procedure (Tang et al., 2021).
A quantity of 10 mg dried sample is dissolved in 20 ml deuterium oxide (D2O), and then mixed with 100 mg N-(3-DiMethylaminopropyl)-N′-ethylcarbodiimide hydrochloride. The pH value of the solution is adjusted to 4.75 by 0.1 M HCl, with stirring for 1 h to accelerate its dissolution. A volume of 20 ml 160 mg/ml NaBD4-D2O solution is added dropwise, keeping the pH value of the mixed solution at 7.0 by 2M HCl-D2O solution. Afterward, keep stirring for 0.5 h. Then the reaction system is stopped by adjusting the pH value to 4.0 with glacial acetic acid. The reaction mixture is dried before dialysis, and lyophilization/redissolution three times, then boric acid would be removed completely by methanol. The dried sample is used for methylation analysis and further NMR analysis.
The assignments of linkages type in polysaccharides are analyzed with GC-MS according to the method of Wang et al. (2020). A quantity of 2–3 mg dried polysaccharide sample is dissolved in anhydrous dimethyl sulfoxide (DMSO), and 20–30 mg dried sodium hydroxide powder is added to the solution, stirring for 2 h at room temperature. The solution is methylated with 1 ml of methyl iodide for 2.5 h in an ice bath, and the methylated polysaccharides are washed with an equal volume of methylene chloride (CH2Cl2) and water three times.
The dried methylated sample is hydrolyzed in 0.6 ml of trifluoroacetate (TFA) at 100°C for 6 h. After the addition of 1 ml distilled water and 15 mg sodium borodeuteride, the reduced polysaccharides are acetylated with 0.5 ml acetic anhydride the hydrolysate was finally acetylated to obtain the partially methylated alditol acetates (PMAAs).
The PMAAs are analyzed using a GC-MS system (Agilent Technology 7890/7000 QQQ), equipped with a Supelco-2330 column (30 m × 0.25 mm, 0.2 μm film thickness) programming from 160°C to 210°C at 2°C/min, and then 210–240°C at 5°C/min (Yin et al., 2012).
The individual peaks of the PMAA and fragmentation patterns are identified by their mass spectra and relative retention time in GC-MS (CCRC Spectral Database—PMAA). The percentage of methylated sugars is estimated as ratios of the peak areas (total ion current).
The zeta potential and hydrodynamic radius of polysaccharides could be measured by Nano-Zetasizer ZS (H. Lin, Zhang, et al., 2021). Zeta potential is a piece of important information for displaying the charged property of polysaccharides, the operation process of zeta potential determination is shown below:
The micromorphology of polysaccharides could be observed using an atomic force microscope, and the detailed measurement process is shown below (Ai, Zhao, et al., 2022; J. Lin et al., 2020).
Before observation, polysaccharide power is dissolved in distilled water and diluted to a concentration of about 3 μg/ml. To reduce the inter-/intra-electrostatic interactions of polysaccharide molecules, the pH value of the polysaccharide solution is adjusted to 2 as usual. Then, 2 μl of polysaccharide solution is uniformly sprayed on a freshly cleaved mica sheet which is pasted on a round iron sheet and put in a culture dish, and then, the culture dish is sealed by plastic wrap which is pricked few holes, and air dried at 30°C for 30 min. After air drying, the mica sheet is scanned in tapping mode using a P-S10 probe made of silicon nitride. The obtained data are processed into two-dimensional (2D) and three-dimensional (3D) images by the Nanoscope Analysis software (Bruker). After the software processing, an image (Figure 3) of the atomic force microscope would be obtained as below.
According to the ruler in the image and the data in the software, the morphology, length, height and other information of the polysaccharide could be observed. Notably, polysaccharide presents as linear or branched conformation under an atomic force microscope and possesses a height of 0.1–1 nm in general, which could be a criterion for judging whether the polysaccharide molecules are aggregated (Ai, Meng, et al., 2022).
The functional groups in polysaccharides could be determined by Fourier transform infrared spectrometer. The determination process is shown below: Five milligrams of polysaccharide powder is mixed with 0.5 g dried KBr, and the mixture is ground in an agate mortar to pass a 200 mesh screen. Then, the mixture is tabletted as a round piece for determination. Notably, before measurements, the sample mixed, ground, and tabletted process is operated with the baking of an infrared lamp. Distinguishingly, the freeze-dried polysaccharide could be tabletted into a thin piece and determined using an FT-IR spectrometer which is equipped with a Universal ATR sampling accessory, and performed at the attenuated total reflectance mode. FTIR spectra ranging from 4000 to 400 cm−1 are measured and recorded in the transmission mode with a resolution of 4 cm−1. Generally, the common characteristic absorption peaks of polysaccharides are shown in Table 2.
Table 2. The common FTIR peaks assignment of polysaccharides (Ai, Meng, et al., 2022; X. Shi et al., 2021; Ouyang et al., 2020)Absorption peaks (cm−1)Peak assignment3200–3600–OH stretching, intramolecular H bond2800–3000C–H stretching of the methyl and methylene groups, intermolecular H bond stretching1730–1760Esterified carboxyl groups1600–1630Free carboxyl groupsAround 1370The symmetrical stretching of the esterified –CH3 groupAround 1250the stretch vibration of carbonyl –C = OAround 1150C–O–C in the sugar ring1000–1100C–O–H variable angle vibrationAround 900The vibration bands of the pyranoid ring
For polysaccharides investigation by solution-state NMR, the preparation is critical. Most important, the “pure” polysaccharide is highly recommended for the determination (Wang, Zhang, et al., 2021). Subsequently, protons in polysaccharides needed to be exchanged into deuterium atoms usually by solubilization-lyophilization in D2O or DMSO-d6 three times (Guo et al., 2018; Xing et al., 2017). It is then redissolved in a deuterated reagent (such as D2O or DMSO-d6), and then transferred into an NMR tube before testing (Hanfland et al., 1981).
NMR experiments are conducted on an NMR spectrometer, and the sensitivity of the NMR spectrometer may be higher if combined with a liquid nitrogen cryogenic probe. The 1H, 13C, homonuclear correlated spectroscopy (COSY), total correlated spectroscopy (TOCSY), nuclear Overhauser effect spectroscopy (NOESY), heteronuclear single-quantum coherence (HSQC), and heteronuclear multiple-bond correlation (HMBC) spectra are recorded using the standard Bruker pulse sequence at room temperature. Figure 3
1D NMR (1H and 13C NMR), is commonly used in polysaccharide characterization. The α- or β-configuration of an anomeric proton (H1) and anomeric carbon (C1) in sugar residue, as well as some featured signals of groups, is recognizable in both 1H and 13C NMR spectra (Figure 4) (Agrawal et al., 1992; Cui et al., 2005; Plaza & Singh, 2010). Moreover, to avoid the influence of solvent peak, the proton chemical shift of solvent residual peak for H2O and HOD is around 4.79 ppm, and the chemical shifts of proton and carbon for DMSO-d6 are around 2.56 and 39.52 ppm, respectively (Fulmer et al., 2010).
2D NMR technology plays an increasingly important role in the structural analysis of polysaccharides (Figure 5). 1H-1H COSY spectrum contains spin coupling networks, reflecting the correlation of the adjacent proton in a sugar residue (Ernst et al., 1989; Kessler et al., 2010; Shaka & Freeman, 1986), for example, H1 → H2 → H3 → H4 → H5 → H6. While TOCSY, known as homonuclear Hartmann-Hahn spectroscopy (HOHAHA), provides the total correlation of protons within one sugar ring through a J-network (Agrawal et al.,1992), which is identified by signals on the same horizontal or vertical line (Morris et al., 1990). HSQC or heteronuclear single-quantum correlated spectroscopy (HMQC) indicates the correlations between proton and the directly linked carbon (Morssing Vilén, 2013; H. Zhang et al., 2012), especially the anomeric connections are well separated. To figure out the sequences of sugar residues in polysaccharides, NOESY or HMBC is preferred (Guo et al., 2018; Saurí et al., 2015). NOSEY spectrum can be applied to figure out the both intra-residual and inter-residual cross peaks (Cui et al., 2005; Guo et al., 2018). Meanwhile, HMBC spectrum reflects the linkages between adjacent sugar residues, about two or three bonds distance between protons and carbons.
Take a water-soluble heteroglycan (SACP-80) extracted from Agrocybe cylindraceaas an example (Wang, Zhang, et al., 2021). Monosaccharide analysis showed that SACP-80 contained mostly gluctose (44.5%), galactose (20.5%), and a small amount of fucose (5.4%). Methylation analysis indicated that SACP-80 was mostly composed of →6)-Glcp-(1→, a small amount of →3)-Glcp-(1→, →3,6)-Glcp-(1→ and Glcp-(1→ with a total proportion of 61.2%, while the galactose residues were analyzed as →6)-Galp-(1→ and →2,6)-Galp-(1→ with a total proportion of 29%, and the proportion of Fucp-(1→ residue was 9.8%. Finally, more information about the primary structure of SACP-80 was shown by 1D/2D NMR spectra (Figure 6). Five signals occurred at 4.99, 4.87, 4.95, 4.65, and 4.40 ppm and were labeled as residue a, b, c, d, and e, successively (Figure 6A). Likewise, there were five anomeric carbons in the 13C NMR spectrum (Figure 6B). Combined with the anomeric regions in Figure 6D, their corresponding carbon signals were obviously found. For residues, the chemical shifts of H2–H6 were obtained from the cross peaks in the COSY (Figure 6C) and TOCSY (Figure 6E) experiments, while the corresponding signals of carbons were clearly observed from the HSQC spectrum (Figure 6D). The sequence of all residues was determined by the NOESY (Figure 6F) and HMBC (Figure 6G) spectra that clear correlation of residue e between H1 and C6, as well as H2–C1, was found, indicating that it was directly linked to each other. Figure 6Falso showed the cross-peaks between H1 of residue c and H6 of residue e', H1 of residue d and H2 of residue d, H1 of residue d and H2 of residue d, H1 of residue d and H3 of residue d, H1 of residue b and H6 of residue b, as well as H1 of residue b and H6 of residue c, which reflected inter-residual contacts. Combined with the results of methylation analysis and prior relevant literature, indicated that SACP-80 consisted of α-(1→6)-galactopyranan backbone with terminal α-fucosyl units or β-glucosyl units on O−2 position and a β-(1→6)-glucopyranan, which was shown in Figure 7. Overall, the ratio of β-(1→6)-glucopyranosyl, α-(1→6)-galactopyranosyl, and α-(1→2,6)-glucopyranosyl residues was 3:1:1 (Table 3), in accordance with the ratios of monosaccharide composition.
Table 3. Linkage patterns of SACP-20 from A. cylindraceae obtained by methylation and GC-MS analysisMass fragments (m/z)Linkage typeRelative percentage of peak area (%)a43, 89, 101, 102, 115, 118, 131, 162, 175Fucp-(1→9.8 ± 1.4Fucose9.843, 71, 87, 102, 118, 129, 145, 161, 205Glcp-(1→10.6 ± 0.843, 87, 101, 118, 129, 161, 234, 277→3)-Glcp-(1→4.8 ± 0.243, 87, 102, 118, 131, 142, 173, 233→6)-Glcp-(1→40.6 ± 2.743, 87, 118, 129, 189, 234, 305→3,6)-Glcp-(1→5.2 ± 1.0Glucose61.243, 59, 87, 99, 118, 129, 162, 173, 189, 233→6)-Galp-(1→13.2 ± 1.243, 60, 74, 87, 100, 129, 130, 189, 190→2,6)-Galp-(1→15.8 ± 2.4Galactose29.0
Polysaccharides possess various functions and could be utilized as a dietary supplement or functional food for hypoglycemic, antioxidant, and anticancer. In addition, due to their macromolecule nature, polysaccharides also could be applied as food-grade material, such as packaging, film, emulsifier, gel agent, and thickeners. The extraction, purification, and characterization processes of polysaccharides are important prerequisites for their application in the food industry. Although the extraction, purification, and characterization processes of polysaccharides have been studied for a long time, a general process suiting for different polysaccharides is hard to be developed due to the different physicochemical properties of polysaccharides. Therefore, the extraction, purification, and characterization processes of polysaccharides should be further studied for understanding the relationship between the properties and activities of polysaccharides in depth.
This work was supported by the Key R&D Program of Jiangxi Province (20192BBF60043).
The authors declare no conflicts of interest.
Xiaojun Huang: writing – original draft; formal analysis; project administration. Chao Ai: Writing – Review & Editing. Haoyingye Yao: Writing. Chengang Zhao: Writing. Chunhong Xiang: Writing – Review. Tao Hong: Resources. Jianbo Xiao: Conceptualization; Supervision.
None declared.